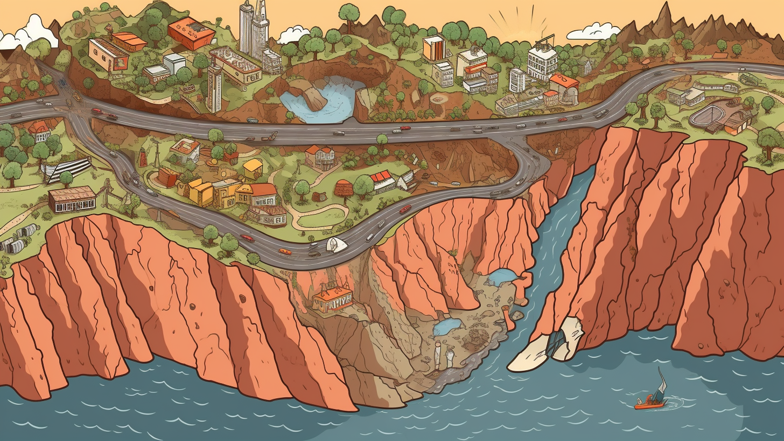
The surface of our planet is constantly changing, with mountains, valleys, and oceans forming and disappearing over millions of years. One of the driving forces behind these changes is the movement of tectonic plates, large slabs of rock that make up the Earth's outer crust. When these plates collide, pull apart, or slide past each other, they can cause earthquakes, volcanic eruptions, and other geological events. In this article, we will delve into the complex and interconnected nature of tectonic plates and earthquakes, exploring how they shape our world and affect our lives.
The Basics of Plate Tectonics
The idea that the Earth's crust is divided into plates that move around was first proposed in the early 20th century by a German meteorologist named Alfred Wegener. He noticed dramatic similarities between the shapes of the coastlines of Africa and South America, suggesting that they were once connected. From this observation, he concluded that the continents must have drifted apart over time, moving to their current positions on the Earth's surface.
Wegener's theory of continental drift was eventually incorporated into the broader concept of plate tectonics, which describes how the Earth's lithosphere - its rigid outer layer - is divided into about a dozen large plates and several smaller ones. These plates are in constant motion, moving at a few centimeters per year relative to one another. The borders where plates meet are called plate boundaries or fault lines.
There are three major types of plate boundaries, each with its own distinct features and geological effects:
1. Divergent Boundaries - These occur where two plates are pulling apart from each other, creating new lithosphere at the boundary zone. An example of this is the Mid-Atlantic Ridge, a vast underwater mountain range that runs down the center of the Atlantic Ocean. As the two plates on either side of this ridge move apart, magma rises up to fill the gap, solidifying into new crust.
2. Convergent Boundaries - These occur where two plates are colliding with each other, with one typically sliding beneath the other. This process is called subduction, and it can create deep-sea trenches, volcanic arcs, and mountain ranges. For instance, the sinking of the Pacific Plate beneath the North American Plate has created the Cascade Range in the western United States.
3. Transform Boundaries - These occur where two plates are sliding past each other horizontally, like cars on opposite sides of a highway. These types of boundaries are often associated with earthquakes, as the plates may get "stuck" and then abruptly snap free, releasing huge amounts of energy. The San Andreas Fault in California is perhaps the most famous example of a transform boundary.
How Plate Tectonics Creates Earthquakes
Earthquakes are one of the most visible and destructive manifestations of plate tectonics. When plates move against each other, they build up stress along the fault line where they meet. This stress can be released suddenly and violently, causing the ground to shake and sometimes rupture.
The magnitude of an earthquake is measured on the Richter scale, which ranges from 1 to 10 and measures the intensity of the seismic waves produced by the quake. Small-scale earthquakes of magnitude 2 or 3 are relatively common near plate boundaries and may only be felt by seismometers. However, larger earthquakes of magnitude 8 or higher can cause widespread damage and loss of life.
The location of an earthquake can be determined by triangulating the arrival time of the seismic waves at different monitoring stations around the world. This information is then used to create a seismic map, showing the epicenter and depth of the quake.
The Impacts of Earthquakes on Society
Earthquakes can have far-reaching impacts on human society, both in terms of physical infrastructure and psychological trauma. Large earthquakes can cause buildings to collapse, bridges to fall, and roads to buckle, not to mention the fires, floods, and landslides that may result. In densely populated areas, the loss of life and suffering can be tremendous, as seen in recent earthquakes in Nepal, Haiti, and Japan.
Aside from the direct physical damage caused by earthquakes, there are also long-term psychological effects. People who experience a large earthquake may feel anxiety and fear for weeks or even months afterward, which can have a negative impact on their mental health and well-being.
Predicting and Mitigating the Effects of Earthquakes
Because earthquakes are such a significant hazard, scientists and engineers around the world are constantly working to better predict, prepare for, and mitigate the effects of these phenomena. There are several tools available to help people anticipate earthquakes, such as GPS monitoring, satellite remote sensing, and ground-based sensors that can detect subtle changes in the Earth's crust.
Another approach to mitigating the effects of earthquakes is to design buildings and infrastructure that can better withstand shaking. Engineers can use materials such as reinforced concrete, steel, and flexible isolation systems to make buildings more resistant to collapse.
Conclusion
The movement of tectonic plates may seem like a remote and abstract concept, but it has profound implications for our world and our lives. By understanding how these plates interact and cause earthquakes, we can better anticipate and respond to these natural phenomena. Moreover, the ongoing evolution of our planet offers a fascinating window into the deep history of the Earth and the forces that continue to shape it today. As scientists continue to explore these topics, we can look forward to even greater insights into the complex and interconnected nature of our planet's crust.
Comments
Post a Comment